We employ and develop different experimental techniques to explore quantum transport through single molecules, nanoparticles, graphene nanoribbons, biological nanowires and confined structures in 2D materials.
The techniques involve the use of mechanically controlled break junctions, electromigrated break junctions and direct e-beam writing for sub-10 electrode spacings. Some of the current topics of interest include:
Quantum interference in single-molecule junctions (Riccardo, Sebastiaan)
Quantum interference plays an important role in charge transport through single-molecule junctions, even at room temperature. Of special interest is the measurement of the destructive quantum interference dip itself. We use the mechanosensitivity of specific molecules to reconstruct the destructive quantum interference dip of conductance versus displacement. Calculations that
include electrode distance and energy alignment variations explain the observations
quantitatively, emphasizing the crucial role of thermal fluctuations for
measurements under ambient conditions. Furthermore, quantum interference can be used to create switches with well-defined conductance states, of interest for neuromorphic applications.
Papers: paper Sebastiaan, Nat. Commun. Xx (2024)
Project (Funding): TUDelft
Collaborations: Marcel Mayor (Basel), Cina Foroutan-Nejad (Warsaw)
Molecular spintronics: spin switching (Serhii, Riccardo, Tristan)
Magnetic fingerprints of the molecules manifest themselves typically at low temperatures by e.g. zero-bias peaks in the differential conductance spectra attributed to Kondo resonances arising and spin-flip inelastic electron tunnelling spectroscopy (IETS) steps.
Different spin systems are under study: all-organic mono- and diradicals, spin crossover components (molecules and nanoparticles) and single molecule magnets. Of special interest is the mechanical manipulation and electrostatic gating of the spin state of the molecule (spin switching).
Papers: T.Y. Baum, S. Fernandez, D. Peña and H.S.J. van der Zant. Magnetic fingerprints in an all-organic radical molecular break junction , Nano Letters 22 (2022)
Project (Funding): Spring (EU project)
Collaborations: Marcel Mayor (Basel), Talah Mallah (Paris), Abhishake Mondal (Bangalore), Eugenio Coronado (Valencia), Diego Peña (San Sebastian)
Quantum thermopower (Serhii)
By subjecting a nanoobject such as a molecule to a temperature gradient,
a current will flow through it without the application of a bias voltage.
This thermocurrent contains important information about the nanoobject under study
such as the spin entropy and universal signatures of Kondo physics. Of special interest
therefore are high-spin molecules such as single-molecule magnets.
Furthermore, by connecting in series to a load resistance, a particle exchange heat engine is created.
Such an engine does not have any moving parts and is a few nanometer in size.
It emerges as an ideal candidate for low-temperature energy harvesting applications where miniaturization is of paramount importance.
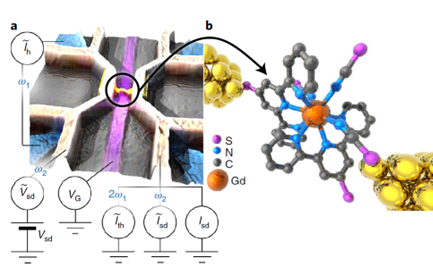
Papers: P. Gehring, H.S.J. van der Zant, et al., Complete mapping of the thermoelectric properties of a single molecule,Nature Nanotechnology 16 (2021)
A. Canetta, S. Volosheniuk, et al. Impact of Spin-Entropy on the Thermoelectric Properties of a 2D Magnet. Nano Letters 24 (2024) 24 (22)
Project (Funding): TU Delft
Collaborations: Pascal Gehring (Louvain-la-Neuve), Michael Perrin (EMPA), Lapo Bogani (Florence), Abhishake Mondal (Bangalore)
Contacting graphene nanoribbons (Yongqing):
Part of the focus of the van der Zant Lab is in contacting and measuring electronic transport through atomically precise graphene nanoribbons.
These carbon-based conductors offer promising prospects for future electronics and spintronics applications. An exciting part of atomically precise graphene nanoribbons is the ability to manipulate their structure, which allows for making metallic and magnetic graphene nanoribbons or ribbons with controlled defects for use as single-photon emitters.
We also study graphene nanoribbons by coupling them to superconducting electrodes.
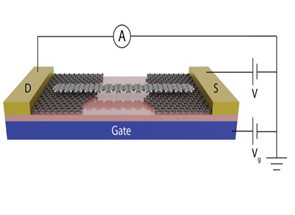
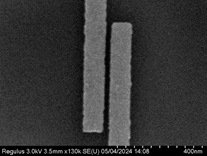
Papers: D. Bouwmeester et al., MoRe Electrodes with 10 nm Nanogaps for Electrical Contact to Atomically Precise Nanoribbons. ACS Appl. Nano Mater. (2023)
Project (Funding): Atypical (EU Pathfinder)
Collaborations: Michael Perrin and Gabriela Borin Barin (EMPA)
Biologically inspired charge transport (Cosimo):
We study transport through a variety of biological systems including single amino acids, peptides, ferritin particles and biological nanowires.
Especially the latter have captured our attention recently.
Specifically, multicellular cable bacteria display an exceptional form of biological conduction sustaining electrical currents across centimeter distances through a regular network of protein fibers embedded in the cell envelope.
The fiber conductivity is among the highest recorded for biomaterials, providing a promising outlook for the emerging field of bio-based electronics, but the underlying mechanism of electron transport remains elusive.
Low-temperature measurements indicate that quantum effects can manifest themselves in biological systems, revealing a high degree of spatial ordering in their fundamental conducting structures.
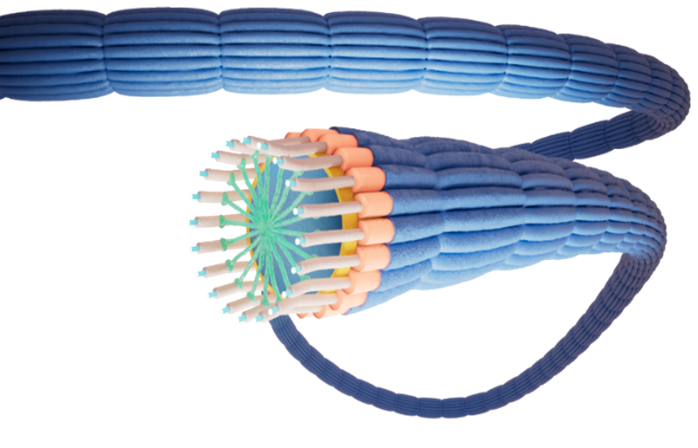
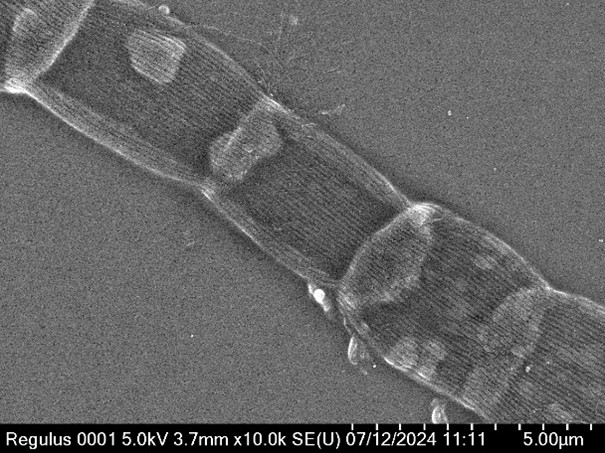
Papers: J.A. Labra-Muñoz and H.S.J. van der Zant, J. Ferritin single-electron transistor, Phys. Chem. B 128 (2024);
J.R. van der Veen, et al. Temperature-Dependent Characterization of Long-Range Conduction in Conductive Protein Fibers of Cable Bacteria, ACS Nano (2024).
J.R. van der Veen, et al. Temperature-Dependent Characterization of Long-Range Conduction in Conductive Protein Fibers of Cable Bacteria, ACS Nano (2024).
Project (Funding): PRINGLE (EU Pathfinder project)
Collaborations: Filip Meysman (Antwerp)
Spin and charge Transport in Graphene-Based Heterostructures (Talieh, Josep)
The properties of graphene can be enriched by the proximity to other two-dimensional (2D) materials in van der Waals heterostructures.
We study quantum transport in graphene which is magnetized by the neighboring 2D magnet.
The transport measurements provide evidence for the spin Hall effect and modified graphene band structure which unlocks its potential for future quantum-coherent spintronic and valleytronic devices.
Bilayer graphene (BLG) is a zero-bandgap semimetal that can be gapped under the application of a perpendicular electric field. We exploit this effect to create quantum point contacts and employ the spin and valley degree of freedom present in BLG to create devices with demonstrating specular electron focusing and valley-resolved ballistic electron transport.
Bilayer graphene (BLG) is a zero-bandgap semimetal that can be gapped under the application of a perpendicular electric field. We exploit this effect to create quantum point contacts and employ the spin and valley degree of freedom present in BLG to create devices with demonstrating specular electron focusing and valley-resolved ballistic electron transport.
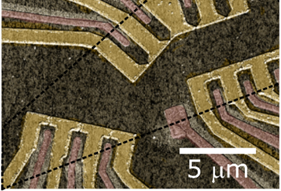
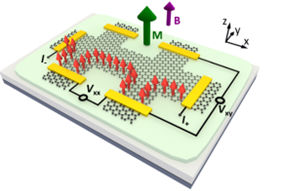
Papers: T. Ghiasi, et al. Quantum Anomalous Hall and Spin Hall Effects in Magnetic Graphene. Arxiv (2024)
J. Ingla-Aynés, et al., Specular electron Focusing between Gate Defined Quantum Point Contacts in Bilayer Graphene. Nanoletters (2023)
J. Ingla-Aynés, et al., Specular electron Focusing between Gate Defined Quantum Point Contacts in Bilayer Graphene. Nanoletters (2023)
Collaborations: Eugenio Coronado (Valencia), Quantum Tinkerer (Delft)